Patrick Meade, Muon Collider Salesman
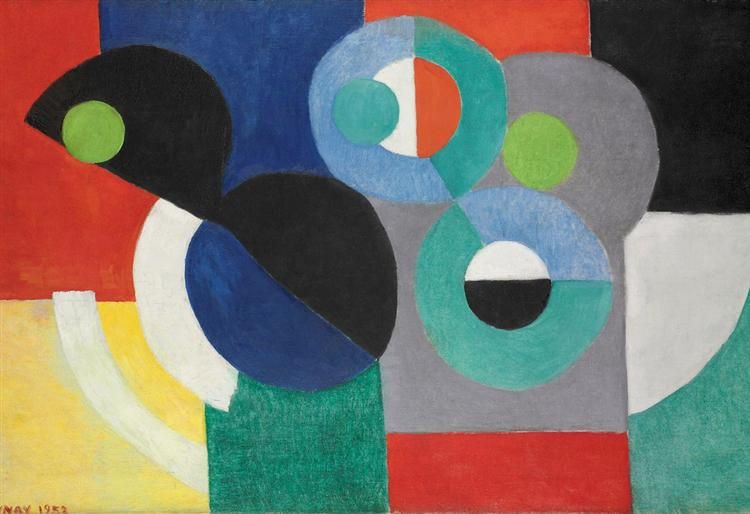
"We need two colliders: an e+e- Higgs factory and a 100 TeV proton proton collider. But if you had a high energy lepton collider, it could really do both for you. It could be the all-in-one machine."
Back when muon colliders were gaining traction during Snowmass 2021 (RIP), I asked for a sales pitch from one of its biggest proponents, Patrick Meade. A professor at Stony Brook University, Patrick enjoys golf, Absolute Bagels, and the newly revamped LaGuardia Airport. In his spare time he works on BSM phenomenology and theory.
(Note: This interview was conducted 4/12/21. It has been edited for clarity and concision.)
Dan Garisto: You're a coauthor on the recently published Muon Smasher's Guide. Why do you want to smash muons together?
Patrick Meade: A muon collider is a technological leap that would allow us to hopefully access energies that are not accessible without having to build gigantic projects like the 100 kilometer FCC-ee/hh proposed by CERN, or the similar experiment proposed in China, the CEPC/SPPC. So in and of itself, regardless of whether anything's screwy with muons in terms of BSM physics, it's just an interesting technological idea.
Why did we write this Muon Smasher’s guide? The last Snowmass process, which is this 10-year exercise for the field to all come together and decide what the priorities are, was dominated, for the energy frontier, by this 100 TeV proton-proton idea where we would just scale up the LHC and reach another order of magnitude in energy. A separate project was always: well, we found the Higgs, it's the most important particle in the Standard Model. We better study it to death, so we should create Higgs factories.
So that was the focus of everybody back then. It's just been a waiting game. CERN said, ‘Well, we're very interested in this.’ Then the European Strategy Report came out, they said, ‘Look, this is our priority, but we don't know if we can swing it financially, so we're going to do five years of cost feasibility studies.’ This time Snowmass came around, and we said, ‘Let's do this from the other direction.’ What could we actually do this time to figure out if there are physics questions you want answered? Instead of some experimentalist or Nima Arkani-Hamed coming to you and saying, ‘Oh, here's 100 TeV, because we pulled this number out of the hat.’
The muon collider is very exciting from the experimental and accelerator perspective, because it's new and it could be possibly done on a much smaller scale. From the theoretical side, since we can possibly access higher energies, the question was: What do we need to do to answer certain questions? So instead of being part of like a big collaboration—there is an international muon collaboration that's kind of based in Europe—we just said, ‘Let's bring back the band from last Snowmass and try to figure out what we could do with it.’ That was the philosophy behind this. It wasn't connected to any anomalies whatsoever. It was just trying to figure out if you really wanted to pitch a project for the field that would give you the most bang for the buck in terms of the energy frontier. The irony is that Snowmass killed the last muon collider.
Last time, the focus was this e+e- Higgs factory and this 100 TeV proton proton machine. There were a few muon collider people. The accelerator physicists were leading this, they were saying, 'Look, we're doing all these cool things that we already know how to do the other things in accelerator physics, Let's try something new.' But there were very few experimental and theoretical physicists saying 'Well, what do we do with a muon collider?' And the whole pitch last time was 'Let's make a Higgs factory with it.' But that Higgs factory was an order of magnitude less impressive than the other ones that were proposed in 2013. This time around, the whole community has changed focus.
DG: There are some interesting sociological questions I want to ask about theorists leading accelerator physicists. But first I want to talk about e+e- and proton-proton because these are such well-established techniques. If it ain't broke, why fix it?
PM: Well, the classic thing is just the scaling, right? I think we've seen over the last 10 years, there's obviously a bit of feet-dragging and imagining in pushing on the energy frontier. Because if the only thing we can do to go to the next step is continually make these things an order of magnitude bigger and bigger and bigger, the dollar amount goes up and goes up, and it eventually becomes unpalatable. I think at some level these are always false dichotomies, because of all these studies about return on investment. It's not like when you build a billion dollar collider we are just taking a pile of money and burning it. It all comes back with technology, it's paying salaries that then go back in the economy. It's not just building some new monument to Bitcoin or something.
DG: A collider to nowhere.
PM: Exactly. So I think that's a bit of why people worried about e+e-. And I don't particularly care so much as a theorist, what the experiment actually is. In Snowmass, I'm one of the conveners for the BSM-Higgs, and all the conveners are paired up—theorists with experimentalists in the energy frontier. My co-convener is Isobel Ojalvo and it was interesting hearing her perspective on these things. From the experimental perspective, it's like, ‘Well, yeah, e+e-, pp are not broke, don't fix it,’—as you said. But at the same time, if you really want to think about energizing the next generation, the grad students, the undergrads, it's much more interesting to say, 'Look, here's something we had to figure out that's new,' versus ‘Let's just keep doing the exact same thing and make it a little bit bigger.' So my community, I think, is not as wed to muons versus electrons with protons. We just want the most energy, we want to find out if there's something underlying nature in a new way. But for experimentalists, and for accelerator physicists, I think it really matters, attacking a new frontier.
DG: Pretend that I'm a government bureaucrat, and I know a little something about high energy physics. I know that for an e+e- collider, one of the things you want is some multiple of the Higgs mass so that you can look for couplings the Higgs has to other particles. You really don't need anything that goes up to 100 TeV. What's the case for going larger with a lepton collider?
PM: Well, I think there's two cases there. The first one, you'd say naively, ‘I don't need much more than getting to the Higgs mass.’ The interesting thing, though, is some of the things that we're not going to have any handle on after the LHC are things like the Higgs’ interactions with itself. And that really is one of the fundamental new properties that the Higgs brings that we haven't measured before experimentally. Additionally, it's not just the energy—you really need a lot of Higgses if you want to search for these rare decays. And unless you can crank up the luminosity by a lot, you run into a problem with the e+e- colliders where you basically need more and more power. You eventually start needing the power of a small country to get more luminosity. But you can get a little bit of growth in the cross sections by going to higher energies. In particular, you can only access multiple Higgs production if you go to higher energies, like the TeV scale or higher—CLIC was proposed for 3 TeV. So just on the Higgs side, there still is a good argument to say 'We need to really get well above the TeV scale to even study the Higgs past order one precision.’ Then the argument of course, from the energy frontier side, is for any new collider, you'd like to have as much energy as possible. You'd like to test nature, go to new distances that we've never seen before.
And the 100 TeV proton-proton colliders do that. 100 TeV sounds really big. But the problem with protons is because they're not fundamental, you're not really getting all that energy. You're only getting a small fraction of that energy. So when you say 100 TeV, you're really accessing the 10 TeV scale at best. If you combine these two statements—’We'd like to study the Higgs more’ and ’We'd like to get to the energy frontier as deep as possible’—a 10 TeV lepton collider immediately brings you to the realm of the 100 TeV proton-proton collider. As I like to keep pitching it, we always said last time, ‘We need two colliders: an e+e- Higgs factory and a 100 TeV proton proton collider.’ But if you had a high energy lepton collider, it could really do both for you. It could be the all-in-one machine. And since it's hard to even build one collider, building two is a lot more difficult sociologically.
DG: Fair enough. Let's talk about the actual physics that's going on here. The two primary processes for looking for new physics and investigating the old stuff to greater precision are muon annihilation and this vector boson fusion. Can you talk about both of them?
PM: Sure. Muon annihilation is basically this idea of using the muons as fundamental particles. We smash them together, and all of their energy gets converted into new particles. But the interesting thing is lepton colliders, even though they're fundamental particles, as we accelerate them to higher and higher energy, there's the possibility that they can radiate photons or gauge bosons, and they start to look a little bit more like a proton. We don't have to think of them as just an incoming electron or an incoming muon. There's a possibility that you have a photon, or W or Z that carries a fraction of the energy. People used to think of just e+e- colliders as fundamental particle colliders. But when you start pushing the energy, you can also start to think of them as having a so-called parton distribution function just like the proton has.
DG: Isn't that bad, though?
PM: It's not bad, because it allows you to access different energies using one collider. The problem that you always face in a high energy machine is that the cross sections are dying one over the energy squared. So you're only sensitive to one energy scale and the cross section is also very small at that energy scale. But these g−2 measurements, where they use BaBar, and they're going to use Belle II in the future, they take an e+e- collision, and they use the fact that you can radiate a photon to effectively get to a lower center of mass energy. So that's why it's actually not a bad thing and lets you cover a larger range both at the highest energies and the smallest energies. So a high energy lepton collider is like this dual-purpose collider: it gets the muon annihilation—or the electron annihilation—it gets you all the energy, but it also lets you do the fun Higgs physics and much lower energies at the same time.
DG: And I guess you still get to avoid a lot of the QCD messiness of gluons. ‘A bag of gluons jumbling around,’ I think, is how people like to talk about it.
PM: Exactly. Whether it's an electron or a muon collider, you don't have any of the ‘brown muck’ as Nathan Isgur first described this QCD stuff that comes along with it. There's interesting new experimental backgrounds, and I mean, people talk about these things. It's a focus of various collaborations. We have a plot in the guide showing what is the ratio of the Higgs cross section to its biggest background at a muon, e+e- collider compared to the proton-proton, and you gain many, many orders of magnitude. So for physics backgrounds, it's just a much cleaner environment.
DG: You call it an ‘aspirational theory case’. How should we be thinking about this in terms of actually planning to put cold, hard cash on the table for an experiment?
PM: We didn't have to tie ourselves to any particular energy. We scanned up to 100 TeV. We're going to try to kind of focus in a little bit more on ‘What does a 10 TeV collider look like?’ Because there's always a bit of a chicken or the egg for muon colliders. As I said, sociologically, last time around was the accelerator frontier leading it. But unlike electron and proton colliders, where everybody knows exactly how to build them, and just scale them up, there's some new phenomena that occur with muon colliders.
In particular, there's a so-called beam-in-beam background where, since muons are not stable particles, they're decaying into electrons and neutrinos. As you get about the point where we’re going to smash them together, some of them are decaying, and there's electrons and neutrinos coming along for the ride, and they'll splash in your detector and make other backgrounds. You need to know not just the detector, you need to know about the whole facility within about 25 meters of the detector on both sides.
There's always been a bit of a chicken or the egg because the experimentalists say, ‘Well, we don't know what the facility looks like, because the accelerator physicists haven't told us, so we can't exactly tell you what the backgrounds were.’ Accelerator physicists say, ‘Well, you haven't told us what energy we're supposed to do. So how do we know how to give you this thing?’ And the theorists say ’We don't care, we just want the highest energy.’ So we're going to try to focus the people interested in this a little bit more to say 'What if we just picked 10 TeV because it's an interesting number for a number of physics cases?' And then we could try maybe within this next year of Snowmass for the accelerator physicists and experimental physicists, all to kind of converge in a design. We can only say the words 'Hey, support a muon collider.'
DG: I’ve reported on muon acceleration techniques before and you need the muon ionization cooling to increase the beam density. [Editor’s note: LEMMA is an alternative, but less popular option.] When I reported on it, they needed to up the factor of cooling by 10,000 to get to reasonable luminosity levels. What are the prospects for increasing that? And when do you think it could be done by? What are the timescales we're looking at here?
PM: At some level, I defer to what I'm told by my accelerator friends, and you get various different numbers out of people. I think the European Community takes a more cautious, slow version of this because the European community also is kind of viewing this as a backup project—FCC doesn't go forwards, we can push all our effort in this direction. And you typically hear that the timescales like 10, 15, 20 years come about for this. The U.S. community, it's been interesting, engaging with them—the accelerator physicists at Fermilab, especially.
The SSC was a big issue for people. And I think they'll give you similarly cautious answers and the order of decades timescales. But I think there's also a feeling that it's really just a matter of how much investment comes in this community. I don't think Snowmass is going to come out and say ‘We recommend to build the 10 TeV muon collider.’ But I think having any significant R&D effort towards building a muon collider that's funded at Fermilab—I mean, those guys developed all this technology. The MICE experiments happened and things are happening in Europe, but Fermilab was really key in this a decade or more ago, and all this was developed. And this is kind of just being kind of rediscovered now and built on a little bit more. So the expertise is there, and the amount of time it takes... I really think it's just a matter of the funding, because nobody really says there's a showstopper. We're trying to get people who are a little bit more worried.
I've heard rumors, there's various accelerator physicists who are a little bit more concerned about developing it, but that's what we're gonna try to keep pushing in this process. We want everybody to show their cards. If you are a proponent of it, one of the accelerator physicists that says you can do this, tell us why you think you can. So we're gonna have a talk next week about what is needed to get to 10 TeV with a muon accelerator full stop, and hopefully, any skeptics will be there as well and say 'Look, this is exactly why you're going to fail.' And I think that's the process that we'll hopefully see play out in the next year or so. Hopefully, P5 will at least support R&D in this. I don't think this time around, there's any chance they're going to greenlight and say 'Build this.' But R&D is really important, because in the last 10 years, that knowledge was kind of lost in the U.S.—it was just kind of preserved at best. And it wasn't until some Europeans started picking it up again, more recently, that it has been redone. So unfortunately, it's not necessarily the sexiest funding, but R&D, well, we really need to have that kind of baseline level to say if you ever want to get to the new technology you have to invest in it.
DG: How do you think it compares to things like energy recovery linacs and plasma wakefield acceleration?
PM: Obviously, plasma wakefield sounds like the coolest thing possible in the sense of like, even muon colliders are very large compared to what the acceleration gradients they talk about for this. Early on the Snowmass process—I think it was last June—there was like the established colliders, the accelerator frontier talked about them. And there's another day where the plasma wakefield people made their pitches, the various different types of wakefields, the muon collider people. And I've tried to engage with the e+e- community as much as possible, but it really hasn't gone forwards exactly as much. There's a lot more serious people working to get to the physics on the muon side than the plasma side.The energy recovery linacs are interesting. But I personally think this kind of at least order 10 TeV scale is the scale where you're really starting to get the interesting things coming out of it and people have proposed making a version of FCC-ee that can maybe get to like 500 or 600 GeV with an ERL to then do more top physics. But unless you really kind of get to this order 10 TeV scale, it's really hard to make a case that you're going very far beyond the LHC.
DG: What are the conversations that you've had with accelerator colleagues already like? Is it more excitement or more caution? I know that people have a chip on their shoulder because the muon program effectively got canceled.
PM: It's just kind of cautious optimism from their side, because this is something that they have that scar tissue leftover from the last Snowmass process. But as I said, it's also very cautious because obviously, if CERN says ‘We're going to build the FCC,’ all of these international or European versions of this are not going to be on the table. I mean, they're going to build the FCC. On the U.S. side, I'd say a year ago or so, it was super cautious, and no one was that enthusiastic. But as Snowmass has gone it's kind of just been slowly increased the entire way. It's been interesting. There's two representatives from the accelerator frontier and they were very interested.
And I think early conversations with people I heard at Fermilab were very pessimistic. Some people were talking about, well, let's resurrect the 125 GeV muon collider version of this. But I think there's slowly been kind of this view of like, if there really is a project of a high energy muon collider, it's different than what we talked about before, it's exciting to all these different communities. And so I'd say the excitement level is just kind of slowly ramping up further and further, and we'll see if g−2 people go crazy after this or not, but I think independent of any of the muon anomalies, I've just seen, slow and steady increase in the number of people that are coming to the meetings increasing as a function of time. So it's really exciting.
DG: All right, well, you brought it up. So let's talk about the muon g−2 anomaly. You can bring in LHCb, if you want to. How does that change everything?
PM: I don't know. We talked about anomalies once before. And I started thinking about all the various anomalies that I've seen in my kind of 20 years of being an active physicist. And it's absolutely crazy how many anomalies there have been. Many that didn't even reach publication. Many of them were literally just, hey, here's some data that was weird at the Tevatron. And then some theorists get brought in and we kind of figure out ‘What could this be?’ There's been even crazier things that got published beyond g−2. It's just the community missed them, because it wasn't hyped in that direction of 'This was going to be a real test of the Standard Model.'
You and other science writers have documented this well. There's a number of different ways to look at anomalies, like you say, 'well, an experiment could have done something wrong,' okay. And it could be something physically wrong, they could have estimated something wrong. There's theory issues where the theorists could have just made the wrong prediction. And so that agreement will change as a function of time. I kind of view g−2, the fact that it agreed so well, with Brookhaven as just a statement that the experiment did an amazing job and this is the best measurement going forward. Then I'm just kind of stuck at the next step of what exactly is the theory prediction?
Since I've seen so many cases at the energy frontier, where you'd see in a channel, they say, 'Oh, this is the cleanest possible channel at the LHC or the Tevatron or something like this, QCD possibly couldn't screw this one up.' And then you wait, and you go to higher orders and you understand things a little bit better. There's even been cases where there's been subtleties of the experimentalists deciding to look at a certain fiducial region of their data. And it seems like just okay, we're just cutting something out in our data. But because we're dealing with quantum mechanics, whenever you kind of look in a certain region, there's quantum mechanical implications on the actual QCD calculations that can show up that I've seen happen at the LHC in a weird way.
So when I see that the white paper came out, and there's this discrepancy, it's certainly interesting. But then you dig the next level down, and you say, ‘Well, where does the white paper say a discrepancy is really driven by?’ It's driven by looking at this e+e- at low energy data into hadrons. And using the optical theorem and relating that to get this hadronic vacuum polarization. And then you say, ‘Well, all these groups have got the same numbers.’ So that's kind of nice. But then you take the next step down, you say, ‘What is the data they used?’ And the data they use is bouncing all over the place.
For instance, the numbers from KLOE and the numbers from BaBar kind of mutually exclude themselves. They can't both be right. And the white paper did a great job in the sense that they did various different combinations. They said, ‘Let's throw out BaBar. Let's throw out KLOE. Let's enlarge the error bars.’ What is the right way to present this as a prediction? If you wanted to do the ultra-conservative view of this, you could have taken what is the thing that agrees best with g−2, the Babar results, and then just use the error bar around there. And now you've gone from something that looks like a four sigma excess to less than a two sigma excess. Now, that's not necessarily right. But if you're really saying new physics is relying on us understanding nonperturbative QCD it makes me a little bit worried given all the other anomalies I've seen QCD screw up when we're supposed to be in the perturbative regime.
DG: That's fair enough. And it makes sense why you wouldn't want to base it off of the g−2 anomaly. But nonetheless, I think people are interested and creatively driven by anomalies. So maybe a better question is: How do you responsibly harness that interest for a muon collider?
PM: I think there's two directions you can go with it. The reason why so many papers came out after the g−2 anomaly, for instance, is because we've been sitting with this for 20 years. This anomaly can point to naively a low scale, order the electroweak scale, people have known all the solutions, and you can kind of just say, ‘Hey, supersymmetry we worked out all these things 20 years ago, so now I'll just push enter on my fit with this new central value.’ and generate 40 papers.
DG: I think it was only 33 the first day.
PM: Yeah, whatever the right number is. But I think it's interesting that from this creative perspective, I don't think I've seen that many new things because people have already hashed this out. They know what are the basic building blocks. The set of people that were trying to think about connecting this anomaly to the muon collider, it's interesting, because they kind of tried to say, 'what is the worst case scenario like?' David and I had looked at this for electroweak baryogenesis over 10 years ago, and he's kind of applied the same philosophy of trying to say like, ‘Could you have 100 TeV particles instead of 100 GeV particles doing this? And what would those assumptions be?’ Which is an interesting way to go about it if the anomaly persists.
But I also flip this around to say if you didn't want to build a case for a new collider, just on an anomaly that you weren't 100% sure on. If you don't couple, just a new singlet to a muon and only a muon and you said, ‘Hey, this could be some new electro-weak charged state’—which people have thought about for the last 20 years. Then this order 10 TeV number, again, is in the right ballpark to investigate all of these solutions. So it's kind of just a nice complementarity aspect of it. We tried to address that as well and not just with g−2. One of the problems of the energy frontier that I tried to express as the tortoise and the hare—there's a lot of low energy experiments that you can do to try to get a really precise number and say, ‘Oh, well, there's a deviation or not.’ Or we have other probes, like gravitational waves, if we see some stochastic gravitational wave background that's above the prediction. Okay, there's a lot of astrophysics in there. It's interesting to ask the question: What sort of scales would you need? So we went through this exercise not with g−2. But with gravitational waves with muon flavor, like the Mu2e experiment. I mean, if they see a deviation there, it's going to point to some ridiculous scale. And so it's important to understand what kind of colliders you might need to get there. Because I think no one's ever gonna believe, from an indirect or precision measurement, that it's 100% new physics, something beyond the Standard Model, until we test it in another way. I think the timescales involved, we're gonna sort that out, before somebody is gonna say, build a muon collider. But even if I didn't want to come up with a crazy theory that just coupled to the muon, and only the muon, for some reason, and I just wanted to say, ‘Look, some generic electroweak particles naturally could explain this.’ The order 10 TeV scale is very interesting from FCC-hh, perspective and from a muon collider's perspective. And so you could definitely make, as an argument for the next kind of scale for the collider, without having to say build a 100 TeV muon collider.
DG: Yeah. I want to go back to the sociology. So last time, you had the accelerator physicists leading the charge. This time you have the theorists. Is that better? Is that worse? How does that change the momentum, if you will? And what do you need from accelerator physicists moving forward?
PM: I think for any individual community to be leading it, it's never going to work. The nice thing about the theory community getting more involved, is it really set out a bunch of targets to why you want to do certain things, or what physics could you access if you get to a certain scale? We always think about that with any experiment, experimentalists could always build their favorite experiments to test some phenomenon. But the whole point is, we know there's the Standard Model, we know there's approximately ΛCDM. And unless you understand how that experiment fits in with everything else, you really can't necessarily get as much excitement from the community to say you're doing something new.
So I think the theorists being involved this time around makes it a much more important case, because there was a lot of leading from that with the 100 TeV collider and thinking about these e+e- Higgs factories. But at the same time, the reason I think that was the focus last time wasn't just the theory community. It was because the experimental community was super engaged with those too. Particle physics has always tried to have this very public process and being very good stewards of asking for order billions of dollars in public funds. Unless you get the full community involved, you're never gonna do it.
Last time, it wasn't that it was a bad thing that the accelerator physicists were leading it. They didn't engage as much with the other communities. And we didn't all come together around that idea last time. And one of the interesting things this time around, too, is for the muon colliders. It also perfectly dovetails into neutrino physics as well. You don't hear that for like FCC-hh or e+e-. This really could be an interesting step where the largest fraction of the particle physics community gets excited about the same thing that I've seen in the last many decades.
DG: Do you have any other pitches for the muon collider that we need to talk about?
PM: I love hadron machines. I love e+e-. I just think this is a particularly exciting one. I think it was Nima that brought it up to me. There's this proposal by Peter McIntyre at Texas A&M, for a 'Collider in the Sea.' Why do we have a problem building future pp colliders? It's because what we're really doing is at the bleeding edge of magnet technology, and these magnets become more and more and more expensive. If you only cared about the physics gain, and not necessarily whether you're doing something new from the accelerator perspective, or the experimental perspective, you would build the biggest ring with the lowest magnetic fields. You need just something you could take off the shelf, that will cost-optimize it. Actually digging the tunnel is a lot of money, and you always have to make the tunnel effectively watertight anyways. FCC is going to be going under a lake. And actually going through the lake is one of the current options. So his idea was essentially make a modular collider that you can just assemble underwater, and then you don't have to dig. And it's a really cool idea. He could reach 500 TeV with a very nice cost. Now the problem is, of course, it's not the mainstream in accelerator physics so it hasn't been vetted, right? I mean, obviously, this could not work at all. He had nice plans—the Gulf of Mexico worked really well in terms of ocean currents and everything.
He was instrumental back in the early days with CERN, getting the designs up to what we could get for the W and the Z discoveries. So this isn't just some crackpot. I'm super excited about anything, if it gets us the highest energies, but I think as a field going forwards, whatever engages all the components of the field is going to give us the best shot, to really make the case to the public at large, that there's something really new and phenomenal that we could do if we invest. So I'm open to all of them. But the muons are cool.
DG: What do you think about the importance of, I guess you could call it ‘stopgap experiments’? ‘Stopgap’ in the sense that there could be a period of several years where there is no new collider doing anything, or no collider at all above a certain energy. Maybe you do want to build the ILC even if the odds of finding some new physics are pretty low, just because you need to keep the next generation of physicists busy.
PM: People argue even with, like the LHC Do we really need to keep running it till we get to three inverse attobarns? Or do you shut it down and start building FCC as soon as possible? I think it's a really important question, especially for accelerator physicists and experimental physicists, because there's a huge amount of knowledge that could just be lost in accelerator physics, and the community at large if there's not kind of the next real technological push.
But I also got to try to find the silver lining for the physics in each one of these. I just agreed to join this new ILC organizational structure as one of the Higgs conveners. I certainly recognize the limitations of the ILC. But there are also interesting things that the ILC could potentially do that a muon collider couldn't do or FCC-hh couldn't do. So I just tried to figure out what are all the various physics cases you can make. And then if anyone really is interested in going forwards, I think we have to make the best out of whatever options we have. Hopefully, it won't come down to some hand-wringing later that we've lost all the accelerator physicists in the world and we don't know how to build the next experiment. Again, I think it's also how the particle physics community needs to approach funding. I had the honor of being chosen to be part of this Fermilab users, group that lobbies Congress every year. And they all try to talk to all the congressional people. particle physics is incredibly good at making the case for what we do and keeping our funding level and possibly increasing by a little bit.
But I think we really need to have some fundamental questions about if we want to really make an order of magnitude jump into nature that gets us beyond just the status quo, we're going to really have to think worldwide as a field. How do we make that case? And instead of just saying like, as long as we fit within the certain budget, or as long as we fit exactly within the DOE requirements but are just like 10% higher and invest by saving over many years. This is something that if it's at the same level of stopgap experiments, like why do you build a stopgap experiment? Because it's cheaper? And could it give us a lot? Well, maybe instead of thinking about that, how do we actually pitch to the community, something that's an order of magnitude larger? We'll see what comes out.
DG: This is all obviously also coming out alongside Biden's presidential budget. I was looking at some analysis of the data, and it is really shocking to see—with certain caveats—just how much lower R&D is as a percent of GDP, compared to the ‘60s where it was, at like 2%. And we're not even at 1%, we're at like .7%. And, there's big increases, I think it was like a 20% increase for NSF. But that is a long way from getting to 2% of GDP. I don't know, maybe the real answer is that the rising tide lifts all boats. And instead of squabbling back and forth with condensed matter folks, just having that unified message of ‘more funding for science’ is the answer.
PM: Yeah, I mean, Paul Grannis is now an emeritus distinguished professor at Stony Brook. And he's always one of the leaders in the international accelerator panels, etc. I remember him telling me , 'You go back and look at the DOE funding data after the SSC cancellation.’ It's not like that money just got pushed somewhere else, that money just disappeared. So if you have a good idea, and the community can push, I think that's the way to go. We've seen other places like the NIH’s budget, obviously historically went above what the DOE increases are. And you can make the case obviously, NIH is more important to everyday lives. But I really think sometimes we get in these silly contests. ‘Oh, what is this $10 billion number the FCC is going to be’ or something like this, and you really look at return on investments. This isn't literally just saying, we're gonna spend $10 billion on this, and it's just gonna disappear. Not only the science benefits you get out of this, but it's always a positive return on investment. So I think the whole community needs to think a little bit more strongly instead of just always cowering behind. ‘Let's keep the status quo, keep our heads down.’ We really need to think to the future and say, ‘We have good ideas.’ If we could explain them better, there's a hope that we could not only get to this next frontier, but we could also explain how well it benefits all of society. It's not just these 1000 physicists doing this thing.