Bryan Ramson wants to put neutrinos on banknotes
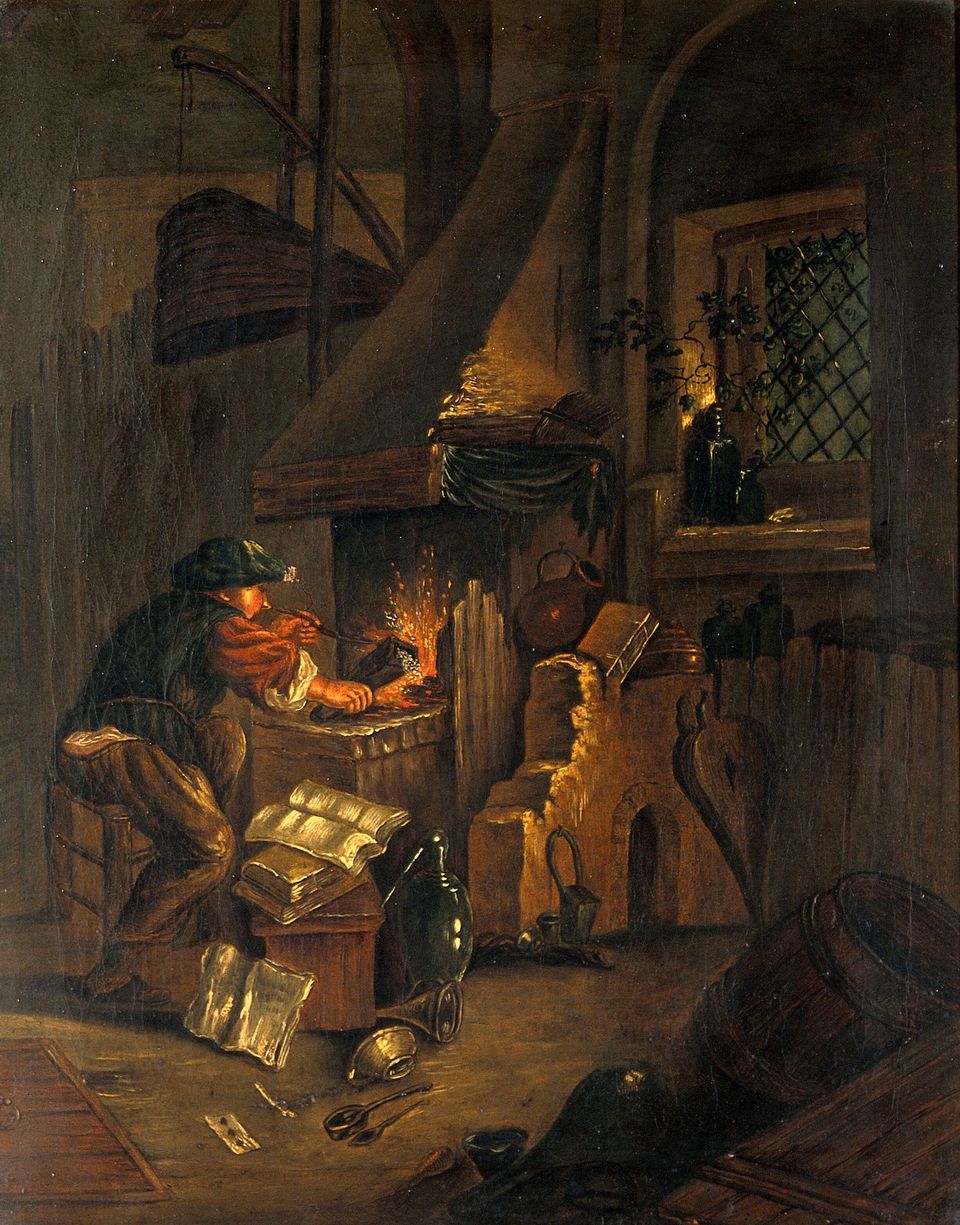
“I've gone essentially from looking at matter-antimatter ratios inside of the proton, to looking at matter-antimatter ratios in the universe.”
Postdocs are arguably the most ignored and most important cog in the machine of academic research. They perform crucial labor, bringing new ideas and contributions wherever they land. As a snapshot into the life of a postdoc, I spoke with Bryan Ramson (who is now an associate scientist at Fermilab) about his path into physics and what it means to spend years in the technical underbelly of an experiment like SeaQuest. From there, we meandered into neutrinos, ethics, and science funding. Bryan works on neutrino physics at DUNE, but he’s passionate about any way to detect neutrinos, honestly. He also likes cats and housing justice.
(Note: This interview was conducted 6/04/21. It has been edited for clarity and concision.)
Dan Garisto: Who are you and what do you do?
Bryan Ramson: My name is Bryan Ramson. I am a postdoctoral researcher at Fermilab. I work on neutrinos. Specifically, I work on the experiments NOvA and DUNE. NOvA is a long baseline neutrino oscillation experiment that's currently running. I look at neutrino-nucleus cross sections. As a graduate student I worked on an experiment called SeaQuest, which looked at the interior structure of the proton. I also am helping to develop the future of neutrino physics in the United States—and that experiment is DUNE.
DG: Why did you get into physics? What led you there?
BR: When I was a kid, I always had a lot of deep questions that I didn't really feel like had really good answers. So I can remember back in high school, asking myself, like, ‘What is fire?’—The funny thing is, I still don't have a good definition of what fire is. Some folks say it's just like an exothermic reaction, right? But it's hard for me to believe that fire by itself is just... that's its definition. I always used to ask myself questions like does light have mass? That turns out to be a rather tricky question. You need a lot of complicated math to really answer it.
DG: The reason you got into physics is because you like to ask questions, or you want the answers to questions?
BR: If you are interested in being any sort of technologist, I think a physics degree is sort of what you should be getting, not only because it gives you a strong technical foundation, but because it trains you to ask questions in such a way that's very analytical. And once you can ask questions in the correct way it's just a matter of finding the answers. I grew up poor in New Orleans. And for me, in order to get out of my material conditions at the time, I always had to think about the future. What are the sorts of things that are going to be happening in the future, that I could get involved in that would get me out of my current situation? I liked computers, so I took a lot of programming classes, and I took some advanced math courses. I realized that physics sort of gives you the underlying math and analytical capability to do both computer programming and engineering and other stuff.
DG: So it's not just asking questions, but something that can actually create a career for you, take you somewhere else, fulfill those kinds of material needs. What are job prospects like right now for a postdoc?
BR: Um, they are interesting. There is the job season that mostly comes up in the late summer, early fall. What you essentially have to do is apply for a bunch of different positions, basically give an academic portfolio, which is your research writing, your summary of your teaching, your summary of your publications, resume, etc. And that takes a lot to prepare, because you basically have to make an entire research plan to say what you're going to do when you get there. On top of that, because of COVID, the actual job prospects have been reduced, by I think like one-third. So I think there are 30% or 40% less jobs than there were this time last year. That being said, I think my prospects are pretty good. I am technically an advanced postdoctoral researcher. So that means I've had about three years of experience. And I am looking at a couple of different national laboratories to either be another postdoc, or looking at universities to switch into a faculty position. If you're asking for prospects outside of physics, those will always be open.
DG: Yeah. The finance community is always happy for somebody who has analytical abilities. There was a tweet the other day that it blamed the 2008 financial crisis on the collapse of the SSC because you had all these physicists who went from particle physics into Wall Street and it was in jest, but there’s a real pipeline there.
So, obviously, the other side of this equation is that there's so much talk about diversity, equity, inclusion. And ‘DEI’ is this thing that industry and universities want. How do you view this kind of diversity, equity, inclusion complex as it relates to your own career, job opportunities, where you see yourself ending up in physics?
BR: Oh, well, that is a terribly loaded question. I'm just going to be honest, because I said I'll always be honest with these things. So I think you called it correctly in the first place, in that it's a complex. What I found in industry and corporations, also in academia and even in government is that there seems to be a lot of discussion about solving a lot of these inequities and things tend to always hit a wall or tend to fall apart, when it comes time to start addressing the material conditions. Because that means that at some point the people who are in power have to open themselves up to not get in the way. I think the greatest example of this is always like when it comes to college admissions, there's a real material consequence to what college you go to and I find that the majority of people who are in positions, go to the best colleges were able to take advantage of those material conditions, and receive the rewards, are significantly less willing to deal with their advantages decreasing. And this tends to cascade throughout the entire structure.
DG: Yeah, it's easy to put a Black Lives Matter sign in front of your house. It's a lot harder to change the zoning and pay for busing.
BR: What I find is that people who are in the best position, who suffer the least from sacrificing some of their privilege, are the most resistant to doing so. So if your kid is economically advantaged, and they are on track to go to Harvard, but your child is not accepted to Harvard because they did not make the cut off because additional students were let in that supposedly are not as well prepared, that student would still go to, I dunno, a very good state school, and probably still have the majority of those advantages. So I just find it interesting, because I think things would be a lot better if people were more interested in an even playing field. And unfortunately, the folks who would have the least to lose tend to be the least interested in that. Now with regard to my job prospects, there is a lot of tokenization happening right now. So I'm in pretty high demand. I think I am the only Black neutrino physicist on the market right now. That puts me in a unique position. I'm getting courted by a few different institutions. Now whether I decide to go depends on how willing they are to actually put some, some real weight behind what they say they can do.
DG: As a white man who really doesn't have to think about how my race impacts me when I'm applying to jobs or positions, I've never been this kind of tokenized quantity. And, in some ways, I feel like that's a relief. It's nice to not think about. But I also haven't suffered discrimination for my skin. So I guess I'm wondering—and maybe this is too personal, so feel free to not answer it—does it feel good to be in demand?
BR: Um, no, not really. I mean, I would much prefer to be courted for what I feel are my scientific contributions. Being honest, it feels like those are somewhat secondary. It's almost like when you go to an amusement park. You have to be this tall to ride the ride. I think as far as my science goes, I'm this tall to ride the ride. But I don't think that's why people are actually trying to attract me. I could be misjudging the situation. I do think I have interesting science ideas. But I don't necessarily know if that's why people are trying to attract me. And I don't think that's something I'll ever be able to know. I can suspect, but there's never an answer either way. And that's sort of where white supremacy and racism sort of live. They always live in the gray area, and the things that you never know. But it is something I think about. I always have to consider what I look like to folks who are different from me. And I don't know if every physicist that I'm competing with has to make that same calculation.
DG: Let's talk about the science.
BR: Good.
DG: Alright, so SeaQuest? Why do we want to learn about the interior of a proton? Who cares what's in there?
BR: It's always good to have basic fundamental knowledge about the universe. You don't know how things can be useful for you. A good example of this is, when the electron was discovered to be a thing that had charge and the use of transistors in computers. There are entire economic sectors that are dependent upon this idea that an electron exists.
DG: Say I buy that argument, that it's important to do that kind of basic research for matter. Why should we care about antimatter in the proton?
BR: So let's define antimatter. Antimatter is actually pretty well understood as part of a complete description of regular matter. The concise definition is it's literally just matter with an opposite electric charge. So an antielectron is literally an electron that is positive instead of negative.
DG: I like that—"part of a complete description."
BR: The reason we care about antimatter in the proton is because the proton is one of the most mysterious physical objects in the universe. It is where most of the mass that we think about as making up everyday matter exists. There’s a common and popular understanding that the Higgs boson is the reason for the mass of particles. And that's true. But if you look at the underlying mass of the particles we know are in the proton, which are three quarks, they only make up about one percent of what we know to be the mass of the proton. The other mass is made up dynamically, right, from the interaction of the quarks with each other.
DG: All sorts of kinematic soup.
BR: Particles are always popping into and out of existence inside of the proton. In order for that to happen and be mathematically consistent, when a particle pops into existence, its antimatter partner also has to pop into existence at the same time. So when you're measuring the antimatter in the proton ball, you're measuring something about the dynamic structure.
DG: Alright, so you've convinced me that we need to study antimatter inside protons. What was your position at SeaQuest? How did you contribute to this research?
BR: So on SeaQuest, I was a graduate student. I had kind of two roles—the number one role was to serve as the lead on the nuclear target. So one of the ways that we learned about the interior of the proton, we basically take two protons and we smash them together. And we need one of those protons to be stationary to maximize the interaction of the beam protons with the stationary protons. We need to have liquid hydrogen hanging out in a secure area. The thing about liquid hydrogen is that it's very dangerous.
DG: I think the Hindenburg could tell me a little bit about that.
BR: Exactly. So my job was to make sure that we had liquid hydrogen basically in a very complicated thermos apparatus. It was cooled to 20 Kelvin. And it was also on a moving table. The reason it was moving was because we wanted to control something known as systematics or uncertainties. So we had to choose between different targets, because in order to actually compare the different flavors of antimatter [Editor’s note: Like anti- up and down quarks.] we had to use two different types of hydrogen. Regular hydrogen, which is just a proton by itself, right? And, deuterium which is a proton bound with an additional neutron.
DG: So to switch between them you move the table?
BR: We move the table, because it's easier to move the table than it is to move the beam.
DG: Oh man.
BR: Beam physics is very difficult. Once you get it right, you don't want to change anything.
DG: I've sat through enough Fermilab meetings where people say, ‘Are the beams not working today? We'll keep trying to get it, back up to performance’ or something like that. That's really funny. I mean, it makes sense. You don't want to move—you can't move the beam, but you can move a table.
BR: Now, imagine this table has two giant thermoses on it. And when I say giant I mean probably the size of a standard refrigerator. That's in a highly radioactive area and it's moving and it needs to be maintained.
DG: Did you write code to move it autonomously?
BR: I came in after most of it was put together. What I had to do is make sure it was kept in a safe operating range, when it's not in the safe operating range that we knew why, and that we were we had control of it at all times. So when I say control I mean it's basically it stayed in the beam. In other words I had to know it was in the liquid phase. We had to know what temperature it was. Because if it changes phase to a gas then there's an expansion problem, right? And then that becomes an explosion hazard. What you do not want is for a highly radioactive area to explode. Because then you're spreading radiation all over the area.
DG: Yeah, I can see why that would be a problem.
BR: I was the apprentice target expert for a year or two. And I became a lead target expert and I maintained it until the experiment turned off.
DG: How close do you end up getting to these targets? And how do you manage the radioactive threat and the threat of explosion?
BR: When you shoot a beam of particles at something, it also becomes radioactive. When we wanted to do work on the target, we had to wait some number of days for the target to not be radioactive. We had to put on all the suits to turn everything off, vent all the hydrogen. Because if you went in there, when the high energy beam was on, it's a big no-no because it would explode. It required a lot of properly scheduling work and having all your ducks in a row and ready to go. And then If anything ever went wrong with it, it was the number one thing that had to be taken care of before anything else could happen. So to answer your question, the way you take care of a nuclear target, that is basically a bomb underground: It's very carefully.
DG: The number of horror stories in physics... The one that always comes to mind for me is the demon core. That's plutonium, which is probably worse than anything you're handling with. But even so. Were you nervous when you were down there?
BR: I was nervous when I first started. And then as I became more comfortable with the system and understood its different states of operation, it was just a matter of making sure things were done carefully. So that was one of the most important things I did. That was like my first job. And my second job was to commission, install, and make sure that it operated correctly, a beam intensity monitor.
DG: Okay, so make sure that there's a certain number of particles in the beam.
BR: Yes, we basically counted the beam bucket by bucket. [Editor's note: Not literal buckets.] And the reason we did that is to calculate the cross section.
DG: Right, you gotta know the flux.
BR: Exactly. The main injector has a variable flux that was incident on our targets. So I was responsible for installing that, doing some initial programming and then from then on, we were able to count how many particles came onto our experiment, and then correlate that with the responses of our detector. That's a very difficult problem. There are many different processes that are happening when you detect something. In order to properly take into account those processes, you need to know how many photons are there in the detector at any given time. That was actually the hardest problem to solve, disentangling the different statistical effects... how the detector responded.
DG: So were you fabricating something? Or was it more of a programming software to detect intensity.
BR: I did not fabricate anything; I got the pieces, put them together. Doing a lot of physics is like Legos. In some cases, you need to ask people to make you special Legos. I installed it as a postdoc and I worked to calibrate it. It was made up of a single PMT. We did a lot of work to make sure that what we were seeing made sense.
DG: Okay, cool. But now you work with neutrinos.
BR: Now I work with neutrinos.
DG: Was there a particular impetus behind the transition?
BR: I had to make a choice if I stayed in physics where I was going to stay. And I wanted to do things that were interesting. I wanted to do things that were taking data. SeaQuest is part of nuclear physics, it's concerned with questions of nucleon structure. Right, there's an entire field that is concerned with those questions, and also has operations at the Jefferson Lab and at Brookhaven National Laboratory. And what's happening right now is that there's a major transition occurring in that field as it prepares for the Electron Ion Collider. It won't be built for another five years.
DG: Yeah, I think it's supposed to start like 2030 or something like that.
BR: It was a question of well, ‘do I want to work on experiments that are operating now, or do I want to wait 10 years for an experiment to come along?’ I decided I wanted to do similar physics, but I did not want to wait for an experiment to come along. Neutrinos have operating experiments now and it turns out they are fixed target experiments. When you have a stationary target you’re shooting protons at—kinematically, it's very similar to neutrino experiments.
DG: Tell me about what you do with NOvA and now DUNE coming up.
BR: So with NOvA, what I've been doing is looking at neutrino-nucleus cross sections. SeaQuest, looked at the cross-section related products of photons hitting stationary protons in hydrogen liquid. What I'm doing on NOvA is looking at products of neutrinos interacting with the molecules that are in the NOvA detector. So cross-sections are like fundamental quantities in particle physics. You have some probabilistic interpretation of how particles are interacting, some theory that describes what's supposed to happen in the Standard Model. The only thing you can really test are cross-sections.
DG: How do you think about cross sections? Do you think about them as a sort of a physical size? Or more of some kind of probability?
BR: I think of it as a probability. And you can think of it as given some amount of flux. What's the probability of a particle of whatever type interacting with a particle of another type? So they're intimately connected to the rate of production of particles.
DG: And for neutrinos, which are obviously, point particles, which have a mean free path through—it's like, a lightyear of lead or something like that.
BR: Six lightyears of lead
DG: How do you think about those kinds of probabilities? They're astronomical.
BR: Well, they're astronomically low. Compared to a proton interacting with another proton, a neutrino interacting with a nucleus… it's very rare. But you still need to be able to measure them to do a lot of experiments. Neutrinos have become such a hot topic because they oscillate as I'm sure you're aware. What that means is that they change flavor depending on how far they travel. right? And the way in which they change flavor, encodes information about or could include information about the prevalence of matter over antimatter. So I've gone essentially from looking at matter-antimatter ratios inside of the proton, to looking at matter-antimatter ratios in the universe.
DG: What kinds of tools are you using to look at this?
BR: So there are a few different simulations. You need a piece of software that basically has all the cross-section calculations in one place, and you can basically turn what you know about the Standard Model theoretically into predictions of particle production rates. That's called a generator. And then you have another sort of simulator, that once you understand what sorts of particles you have, you can grow those particles through a virtual representation of the detector.
DG: Sure, like a Monte Carlo type thing?
BR: Yes, they would both be considered Monte Carlo. They simulate random processes or probabilistic processes. And then we try to make them match with data from experiments, which is possible, without spoiling ourselves in terms of what the answer should be. Then we perform mock measurements on the simulations that we are required to with the data.
DG: How do you handle uncertainties—things we don't know about neutrinos and how they interact? We don't know if it's an inverted hierarchy. We don't know, to precision, θ13, we don't know what δCP is. Neutrinos don't even have mass in the Standard Model unless you add that in. You say you plug the Standard Model in, but what does that actually mean, in terms of what we know about neutrinos?
BR: This is probably one of the largest and biggest questions in all of neutrinos, because there's so much we don't know about neutrinos. And this is actually the case in a lot of physics. What we do is we come up with ways that the Standard Model could be augmented. The short answer is once we come up with these ways, we do as many different sorts of simulations and incorporate these different augmentations. So the problem is, you don't know how closely these models that we come up with that aren't the Standard Model correspond with reality. We come up with as many as we can, we try to do as many different interesting statistical variations as possible. And then we put an estimate on what we're doing given the assumption of x model over y model, right. Now the problem is that leads to bias in your results in ways that are very profound, very hard to understand. So, in neutrinos we do a lot of work trying to be as careful as possible.
DG: When are we going to have our answers on neutrinos? And what are we gonna know by the end of the decade?
BR: That's a hard question to answer. So I did a lot of my postdoc work on protoDUNE actually. The reason that's a hard question to answer is because how quickly we can learn things depends on what the universe has chosen for the things that we're measuring. So our sensitivity to these underlying parameters like δCP, depend on what the values of δCP are. If the value is zero, that means that there's no difference, or there's no CP violation. And the only thing we can do then is try to minimize or try to exclude ever, ever smaller values. And in that case, we would never know. But in our luckiest cases, with full detector use, I think we have δCP to five, sigma in about seven years. And in our unluckiest cases we have I think we have three sigma exclusion for, like 20 percent of δCP values in 15 years.
DG: Okay. That's not so bad. It could be worse.
BR: Yeah, it's not bad. I think DUNE and T2HK are going to be the last generation of these very large neutrino experiments. So I'm really keen to try to get as much out of these experiments as possible, because to make something larger than DUNE... I mean, of course, technology could always improve.
DG: I wrote an article about the liquid argon that's needed for it. It's a significant portion of the entire world's annual production. 70,000 tons is a lot.
BR: I think the stat that got me is that they told me it would take two years to fill up a single module. Two years.That's a scale of preparation for a liquid argon experiment that I am just unfamiliar with. I mean, ProtoDune took a couple months. Even that was daunting.
DG: It's funny, because I think researching neutrinos, as mysterious as they are, you know that what you're doing will be able to detect this unknown. But in other areas, like dark matter, or if you're chasing some kind of supersymmetry model, you don't even know if the search that you're doing will be able to probe that. How do you think about these unknowns in physics?
BR: We're gonna get more controversial.
DG: Alright.
BR: I'm not a theorist. So far be it for me to make, to make statements that should be heated about where we should be going as a science and which theories are better than others. I will say that from my own career, I tend to stay away from these sorts of exotic searches. Because, I mean, at the end of the day, there are things that we actually need to measure that would be useful. I think they are less theoretically exciting but that give us more information about things like nucleon structure. You can somewhat compare it to stamp collecting, I think some people will take offense with that. But you actually do learn about nuclear structure, how things work. It's these fundamental things that you're putting together that will probably allow you to do really interesting things in the future.,
DG: Yeah, the only reason why we're able to do muon g-2 is because we have all the e+e- data that we can use to reconstruct and predict what the hell these virtual hadronic globs are and that's the only way we can make a theory prediction to that kind of precision.
BR: That's an example of the sort of things you can do once you understand the interior of the proton because you know that the majority of mass is created dynamically. The quantum numbers that you need to satisfy are only really attached to the quarks. And once you know where they are, if you wanted to do something like turn off mass, you might be able to figure out how to do that while still preserving the relevant structure of the proton. This is something that seems impossible to do, right. I wouldn't even know how to begin to do it. If you understood to great quantitative detail the structure of the proton. It might be that you find some way to do this in the future.
DG: Some kind of regime where the quarks don't have their couplings?
BR: Well, the coupling is there. But that dynamic portion of the coupling is not there. I mean, of course, it seems absurd, theoretically. You would have to do some really funky math to get that to work.
DG: No, it's interesting to think about. If you actually identify where the mass is coming from, you can think about, at least ways in which that mass is not there. I guess, thinking about different values for the Higgs is kind of the same thing. What if the Higgs vacuum expectation value is different?
BR: So again, that's like super science fiction. But who knows what could happen in 100 years?
DG: Let me ask you about funding. Why are we funding physics? Why are we funding these kinds of ‘out there’ studies, when we could be spending the money elsewhere? You get people who say, ‘Look at the cost of [next big physics experiment]. We could be using this money to feed children.’ There's a lot of responses to that, and I'm not asking you to come up with a response. But I am curious what you think, the social good of physics, and what our responsibility is, given the resources that we have, or we're asking for?
BR: There's so many different responses to that, right? There's one that says, ‘Okay, well science is mostly a good,’ Except for like, where we as human beings where ‘our grasp exceeds our reach’ or something like that.
DG: Something like that, yeah.
BR: Those cases, where we wind up creating things like the nuclear bomb, right, excluding those cases. I think science has been an overwhelming force for good. Given what it has produced, versus how much its cost has always been like a hundred times investment. You always get way more back than you give when you invest in science. And when you invest in your population, they're just so many different knock-on effects.
DG: Sure.
BR: But then when you look at the comparison, things like the military and the Pentagon—a significant portion of our federal budget goes to funding weapons, funding institutions and instruments of war. I'm not sure that the money that is being sent to the military-industrial complex is really giving us a good return on investment.
DG: I think there's a fair number of people who would agree with you.
BR: I mean, how much did it cost to invade Iraq?
DG: A lot. It's like a trillion, I think.
BR: I think space travel is very difficult. I think Mars is an intractable thing to solve. I don't know if we could ever live on Mars, but a trillion dollars is a lot. I think you could figure it out.
DG: What do you think our responsibility is, when we're thinking about taxpayer money, when we're thinking about how we talk to the public and how we try to convince them? I probably shouldn't say this, but NASA's PR department will gin up this big press release every time they find some new traces of frozen water on the moon, or something like that. You can hype up the science to huge levels. You can do all sorts of things to increase public curiosity. Given what you were saying before about the importance of ‘stamp collecting,’ how do you convey that to the public?
BR: I think it's difficult to explain the importance of science to a population that isn't the most scientifically literate. If you look at how science is handled in the United States, to how science is handled in certain countries in Europe—Switzerland has images from the LHC on the money. Yeah, so everybody knows about CERN. Everybody is impressed and happy about CERN. It's not just in Switzerland, right? It's all of Europe considers CERN to be like a real testament to their ingenuity, intelligence-
DG: -and diplomacy-
BR: -and diplomacy. So I think the reason that's the case is because they are more scientifically, on average, they tend to be more scientifically literate than the average American. So we can talk about why that is, but I mean, you have to start there first. You're not going to convince the American public that science is useful until they understand science. You can try to put things in perspective to make 'em understand. But it’s like me telling you that I can build a better mousetrap because I'm always looking to build a better mousetrap. So given that disadvantage, I think it is important to really try to humanize the stories behind the sciences, and sort of make them applicable to everything. A lot of people don't actually know that the internet was developed by many different institutions at the same time. And one of those institutions was CERN because they needed ways to communicate across the computers. (Now, you can also say it was developed at DARPA.) If you go there and see one of the world's first computer servers, I think that's the sort of story you need to be able to tell with your science. That we were trying to understand our universe and in the process we invented the most important stores of human knowledge ever created.
DG: When it comes to the internet, there’s a Simpsons quote I think of. Homer Simpson talks about beer as ‘The source of and solution to all of life's problems.’ But I take your point. Maybe it is intractable until you have a populace that really has scientific literacy. Convincing somebody to let you build a better mousetrap doesn't really work until the person knows what a mouse is, and why you want to build a mousetrap in the first place. You can't just say, ‘Well, my design specifics are better than this previous mousetrap.’
BR: Or even that my mousetrap is better than just getting a gun and shooting the mouse, right? Everybody understands how a gun works.
DG: You'd have a lot of holes in your floor, I think.